|
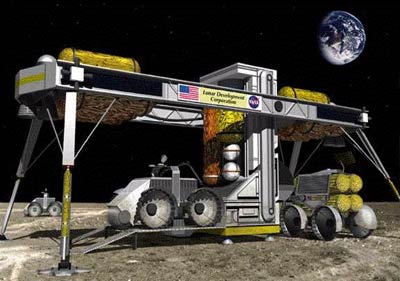 NASA has long considered obtaining resources like oxygen from the lunar regolith key to any future human return to the Moon, but such efforts are more difficult and expensive than simply digging and heating dirt. (credit: NASA) |
The problems with lunar ISRU
<< page 1: utilizing polar ice deposits
A five-step plan for lunar ISRU
A campaign is an end-to-end sequence of missions and programs to accomplish a goal. My view of the first five steps of the required campaign for developing lunar ISRU based on polar ice is as follows:
- The Lunar Reconnaissance Orbiter (LRO) will use a neutron spectrometer to locate hydrogen signals in horizontal spatial pixels of dimension ~ 50 km.
- Despite the fact that neither JSC nor ESAS seem to have the slightest intention of doing this, what is required next is to send several long-distance rovers equipped with dynamic active neutron spectrometers to several of these craters, to cover a few tens of kilometers in each one to determine the local distribution of the hydrogen signal.
- From the results of step 2, a decision can be made as to which specific site (or sites) will be selected for more detailed measurement and verification. The required areal extent of the ice field depends upon the water ice content and the cumulative need. If the outpost requirement is to produce approximately 24 kg/day of O2, this requires 27 kg/day of water (with no losses) and maybe 30 kg/day of water with losses. If we can roughly assume 2% water content in 70% of the top one meter of regolith, then each square meter excavated yields about 1500 kg x 0.7 x 0.02 ~ 20 kg of water. Hence the full-scale outpost ISRU system requires excavating about 1.5 cubic meters per day, processing about 2250 kg of regolith per day, and extracting about 30 kg of water per day. In one year, an area of about 550 square meters is excavated. Over ten years, an area of about 5500 square meters (approximately 75 by 75 meters) is needed.
- We would then send a short-range rover system to the selected site(s) to map out the site with neutron spectrometers in great detail; take subsurface samples to validate rover-mounted dynamic active NS measurements of water-equivalent content; determine the actual form of hydrogen-containing compounds, which are almost surely dominated by water; extract water from some samples and determine the water purity and the potential need for purification; and determine the soil strength and requirements for excavation of the site. In some studies, this step would be implemented with support of a human crew who land in the Lunar Surface Access Module (LSAM). But if in fact step 4 can be done robotically, why would anyone (other than NASA) want to send a crew to do it?
- Develop a one-tenth scale ISRU demonstration system for use at this site, deliver it with human oversight, get it started, and leave it to operate autonomously. In this task, several factors will be challenging:
- Even at one-tenth scale, there is a need to excavate 225 kilograms of regolith per day, transport it to the water extraction unit (WEU), heat the regolith to well over 300 kelvins to drive off water vapor, remove spent regolith from the WEU, dispose of the spent regolith and any dry regolith layer that may lie atop the ice-containing layer, and deal with the 3 kg/day of water produced. If the water is to be electrolyzed and the hydrogen and oxygen stored, that needs to be designed into the system. All of this takes place in the dark at very low temperatures.
- Definition of autonomous operations, including disposal of waste regolith, methods of excavation, and vehicles for transporting regolith to and from the WEU will require a great deal of study and analysis.
- Power is likely to be a major show-stopper at every stage of this enterprise. If the demonstration must run autonomously after the crew leaves, how is it going to get sufficient power? It seems unlikely that enough RTGs will be available. Will NASA develop a nuclear reactor? There is no evidence that it will.
Nevertheless, even if lunar ISRU is not a paying proposition, it still needs to be done effectively. |
It seems clear that neither JSC nor NASA as a whole have given adequate thought to the big picture of lunar ISRU, its requirements, and its benefit/cost ratio for the whole campaign. A sober assessment of the requirements for developing and implementing lunar ISRU compared to the “value” of mass saved, creates significant doubt as to the value of lunar ISRU.
Nevertheless, even if lunar ISRU is not a paying proposition, it still needs to be done effectively, and the approaches taken by JSC and ESAS do not seem to fit that requirement.
Cost analysis for ESAS ISRU
In this section, we compare costs of an outpost with and without ISRU. The following basic assumptions are adopted:
- Costs to develop various vehicles (CEV, LSAM, etc.) are borne by Constellation and do not enter the ISRU vs. non-ISRU comparison.
- The various vehicles used for sorties are also used for outpost deliveries and returns.
- The outpost is operated for 10 years with two exchanges of crew per year.
- A cargo delivery of 32 MT to the lunar surface is made once/year to deliver infrastructure at a cost of $1.2 billion for launch and launch operations.
- LOX-methane propulsion is developed for ascent propulsion whether ISRU is used or not.
- Ascent from the Moon requires 4 MT of oxygen propellant.
- The “gear ratio” (mass in LEO/payload landed on the Moon) for cargo deliveries to the pole is around 4:1.
- The benefit of ISRU is elimination of 4 MT of oxygen ascent propellant, twice per year.
The benefit of ISRU is difficult to assess. Whether ISRU is used or not, there will be two crew deliveries and two crew returns per year. The same vehicles are used whether ISRU is used or not. The only difference is that in the ISRU case, the LSAM can be landed with empty oxygen ascent tanks. Simplistically, it would appear that in the ISRU case, an extra 4 MT of payload can be carried to the lunar surface with each crew delivery (8 MT per year), or alternatively, a rocket booster or two can be removed from the launch vehicle to reduce the initial mass in LEO (IMLEO) by up to 16 MT (4 x 4) for each crew delivery. The increased payload to the lunar surface would acquire value if we assume, as stated in the assumptions above, that periodic (maybe once a year) cargo deliveries are made to the Moon in which no CEV is used and no return is used. It is likely that such a cargo delivery system could deliver perhaps 32 MT to the lunar surface, assuming its IMLEO is about 128 MT. In that case, using ISRU with its annual increase of 8 MT of cargo to the lunar surface in crew deliveries, every fourth cargo delivery would be eliminated by supplying extra payload with each crew delivery. The net saving from use of ISRU is one cargo delivery every four years. If the cost of launching a cargo delivery mission is say, $1.2 billion, the annual saving from use of ISRU is about $300 million.
The cost of an ISRU system includes the following items (not a complete list):
- Development of processing technology. This includes a system that can receive regolith, heat it to drive off water vapor, condense and collect the water, and release spent regolith,
- Development of excavation and regolith transport technology. This includes autonomous systems to excavate regolith, transfer it to a processor, and dispose of spent regolith.
- Simulation and test of systems on Earth. This may involve very large, cold evacuated chambers where simulated field operations can be tested on Earth prior to test on the Moon.
- A nuclear reactor power system must be developed that would not be used except for the fact that the location is in the dark near the south pole.
The development cost for the ISRU components is difficult to estimate. A rough guess is that the development cost for ISRU components will be $800 million. In addition, a wild guess for the nuclear reactor is $5 billion.
Prospecting will likely involve the following stages:
- LRO observation from orbit: $460 million
- Ground truth long distance rovers equipped with neutron spectrometers to locate sites (four missions at approximately $800 million each)
- Ground truth local mission to validate selected site with subsurface access (one mission at $1.2 billion)
Total cost for prospecting: about $5 billion.
Saving $300 million per year would require over 60 years to break even, and it would be worse if we take into account that ISRU investment is up front whereas return on investment is delayed many years. |
Beginning with a one-tenth scale system, and extending this to a larger scale “dress rehearsal,” two significant installations for autonomous ISRU operations need to be developed, delivered, installed, debugged, and set operating on the lunar surface. Since each of these involve sorties operated by human crews, the cost is roughly estimated to be $8 billion for the two demonstrations.
The total cost to implement ISRU is estimated to be:
Development: $6 billion
Prospecting: $5 billion
In Situ Test and Validation: $8 billion
Total: $19 billion
Saving $300 million per year would require over 60 years to break even, and it would be worse if we take into account that ISRU investment is up front whereas return on investment is delayed many years.
A new paradigm for lunar ISRU
The problems in using ISRU within the current lunar architecture include:
- Lunar sorties must be fully capable of landing, ascending, and returning without utilizing ISRU;
- ISRU is tacked on as an afterthought to lunar missions well after outposts are set up;
- Required capabilities for landing, ascending, and returning that must be developed in the beginning without ISRU are not mitigated by later use of ISRU;
- Of all the many masses that must be sent to the Moon, ascent propellants (to lunar orbit) are only one moderate element (~4 metric tons);
- If ISRU is used only to supply propellants for ascent to orbit, the mass benefits are modest, resulting in modest equivalent cost savings;
- The investment needed for prospecting, validation of resources, validation of regolith excavation and handling, and validation of lunar polar ISRU end-to-end system is large;
- If we ignore mass savings from ISRU, and concentrate on return on investment in ISRU, lunar polar ISRU does not pay back the initial investment in the current ESAS architecture;
- This implies that setting up an outpost near the pole has no justification; and
- This, in turn casts doubt on the entire basis for the enterprise of returning to the Moon!
Current NASA plans call for ascent propulsion based on space storable NTO-MMH. If NASA does not replace this with an oxygen-based ascent propulsion system, then even the meager potential benefits from ISRU in the current ISRU architecture (providing ascent LOX) will disappear, and lunar ISRU would have essentially no value.
Given that ISRU does not mesh with the current ESAS architecture, alternative architectures must be considered: either that or eliminate ISRU entirely from the current architecture.
This leaves us with significant mass challenges. To justify retention of ISRU:
- Cryogenic propulsion utilizing LOX and possibly LH2 must be used throughout descent and ascent from the Moon.
- A high-leverage user of ISRU products must be found in addition to ascent propellants.
- Significant reductions in IMLEO must result from use of ISRU.
A very significant potential target is descent propellants, totaling some 20 to 25 MT. In order for ISRU to significantly impact the lunar exploration campaign, the following conditions must be fulfilled:
a) ISRU must be built into the very fabric of the lunar campaign so that all space and launch vehicles are designed and sized to use ISRU from the beginning. (This is as opposed to the ESAS approach of only using ISRU rather late in the campaign as an add-on to a system that does not require ISRU).
b) In order for (a) above to be possible, an extended robotic campaign must precede the human campaign, to establish a working ISRU plant as a fundamental asset for the first human sorties. This will undoubtedly delay return of humans to the Moon by several years.
c) Lunar polar ice must be the ISRU feedstock of choice because it is the only reasonable hope for a workable system.
d) Oxygen must be retained as an ascent propellant. It would also be useful to use hydrogen for ascent as well.
e) Utilization of ISRU products must be expanded to include descent propellants as well as ascent propellants.
Dr. Donald Rapp is a former senior research scientist and division technologist at JPL. He served as manager of the Mars Exploration Technology Program there, studying in situ propellant production, solar power on Mars, and other Mars-related technologies.
|
|